Translate this page into:
Transforming Cancer Treatment: The Evolving Landscape of Targeted α-Particle Therapy
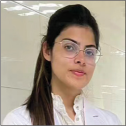
*Corresponding author: Ivin Thomas Jolly, School of International Education, Anhui Medical University, Hefei, Anhui, China. ivinjolly25t@gmail.com
-
Received: ,
Accepted: ,
How to cite this article: Jolly IT, Bhatia S. Transforming Cancer Treatment: The Evolving Landscape of Targeted α-Particle Therapy. Indian Cancer Awareness J. 2024;3:33-7. doi: 10.25259/ICAJ_23_2023
Abstract
Targeted α-particle therapy, a promising innovation in the field of cancer treatment, represents a convergence of groundbreaking science, advanced technology and the potential to revolutionise oncology. This review explores the historical development, mechanisms of action, preclinical and clinical applications, safety considerations and the future directions of targeted α-therapy. We delve into the evolution of this therapy from the early experiments with alpha particles to the synthesis of α-particle-emitting radionuclides, highlighting the key milestones that paved the way for its clinical translation. The mechanisms of action section elucidates the radiobiology of α-particles, focusing on their high linear energy transfer and deoxyribonucleic acid-damaging properties. In addition, we discuss the immunomodulatory effects of α-therapy, offering insights into its broader impact on the immune system’s response to cancer. Preclinical studies, informed by animal models and in vitro experiments, provide essential information on the efficacy and toxicity profiles of α-therapy. Lessons from these studies guide the transition from laboratory success to clinical trials, which are comprehensively reviewed, offering a glimpse into ongoing and completed studies as well as their associated challenges and limitations. Patient selection, diagnostic imaging, and safety considerations are paramount in the clinical translation of targeted α-therapy. We explore personalised treatment approaches, dosimetry calculations and advancements in imaging techniques that aid in treatment planning. Moreover, we discuss future directions, including the potential for combination therapies and non-oncological applications of α-therapy. Finally, regulatory and economic aspects are addressed, recognising the importance of approvals from agencies such as the Food and Drug Administration and international regulatory bodies. Considerations of market dynamics, cost-effectiveness and patient access are crucial in assessing the feasibility and integration of α-therapy into mainstream oncology. In conclusion, targeted α-particle therapy emerges as an evolution in cancer treatment, with the potential to redefine the oncology landscape. To unlock its full potential, multidisciplinary collaboration, ongoing research and dedicated efforts to address safety and regulatory aspects are imperative. This review encapsulates the journey toward establishing targeted α-therapy as a standard of care and sets the stage for the next chapter in the fight against cancer.
Keywords
Cancer evolution
Dynamic decision support systems
Precision medicine
Artificial intelligence machine learning
INTRODUCTION
The treatment landscape for cancer has witnessed a remarkable evolution over the past century. From rudimentary surgical procedures and toxic chemotherapy to the advent of precision medicine, there has been a continuous pursuit of innovative therapies to combat this multifaceted and relentless disease. One such innovation that has garnered significant attention is targeted α-particle therapy, which stands at the precipice of revolutionising the field of oncology.[1] The clinical translation of targeted α-therapy represents a fascinating intersection of groundbreaking science, cutting-edge technology and the potential to redefine the future of cancer treatment.[2] In this review article, we embark on a journey to explore the evolution and revolutionary potential of targeted α-therapy in the context of clinical applications.
BACKGROUND AND SIGNIFICANCE
Cancer remains one of the leading causes of mortality worldwide, with a significant impact on public health and the global economy.[3] Traditional treatment modalities, such as surgery, chemotherapy and radiation therapy, have been the cornerstone of cancer management for decades.[4] While these methods have undoubtedly saved countless lives, they are not without limitations.[5] Chemotherapy, for example, often lacks tumour specificity, leading to widespread toxicity and a range of adverse effects that can significantly impact a patient’s quality of life.[6]
In recent years, the paradigm of cancer therapy has begun to shift toward a more personalised and targeted approach.[7] Precision medicine, with its emphasis on tailoring treatment to an individual’s genetic makeup and the unique characteristics of their tumour, has yielded notable successes. However, this approach primarily revolves around small molecules and monoclonal antibodies, leaving a distinct gap in the therapeutic toolkit.
Targeted α-particle therapy, which harnesses the immense energy and precision of alpha particles for the treatment of cancer, represents a promising approach in oncology. By directing these high-energy particles specifically to cancer cells while minimizing damage to surrounding healthy tissue, this therapeutic strategy holds potential for enhancing treatment efficacy and reducing side effects compared to traditional radiation therapies.[8] Alpha particles are helium nuclei consisting of two protons and two neutrons.[9] They possess remarkable therapeutic properties, including high linear energy transfer (LET) and limited tissue penetration.[10] These characteristics make α-particles exceptionally effective at causing irreparable damage to cancer cells while sparing surrounding healthy tissue. This selectivity is the cornerstone of α-therapy’s potential.
OBJECTIVE OF THE REVIEW
This review aims to provide a comprehensive overview of targeted α-therapy, tracing its historical development, elucidating the mechanisms of action and evaluating its preclinical and clinical applications.[11] We will delve into the intricacies of patient selection, safety considerations and the role of diagnostic imaging in treatment planning. Furthermore, we will explore the future directions and potential of α-therapy in cancer treatment, as well as its applications beyond oncology. Finally, we will discuss the regulatory and economic aspects of bringing this revolutionary therapy to patients worldwide.
HISTORICAL DEVELOPMENT
To appreciate the current state of targeted α-therapy, it is crucial to understand its historical evolution. The use of alpha particles in medicine dates back to the early 20th century when radium-based sources were employed for radiation therapy.[12] While this laid the foundation for future developments, the real breakthroughs came with the discovery and synthesis of α-particle-emitting radionuclides in the mid-20th century.[13] These radionuclides, such as actinium-225 and bismuth-213, opened the door to highly specific α-therapy. Pioneering work by researchers and institutions worldwide paved the way for a new era in cancer treatment.
The clinical translation of targeted α-therapy is a subject of considerable excitement and scientific exploration. As we delve deeper into the pages of this review, we will uncover the mysteries of α-particles, their potential to revolutionise cancer therapy and the challenges that must be surmounted for this revolutionary approach to become a standard of care. From historical breakthroughs to current clinical applications and future horizons, we will explore the multifaceted landscape of targeted α-therapy, seeking to answer the question: Is it an evolution or a revolution in the field of oncology?
Historical development
The section on historical development traces the origins of targeted α-therapy and the pivotal milestones that have shaped its evolution. It explores the early use of alpha particles in radiation therapy and how radionuclides such as actinium-225 and bismuth-213 were discovered and synthesised, laying the groundwork for targeted α-therapy. Pioneering researchers and institutions played a crucial role in developing the concepts and techniques that underpin this therapy. By understanding this historical context, readers gain insight into the scientific journey that has brought us to the cusp of a revolution in cancer treatment.
Targeted α-emitters
This section provides an in-depth look at the α-particle-emitting radionuclides used in targeted α-therapy. It highlights their characteristics, selection criteria and availability. Readers will gain an understanding of the distinct properties of different radionuclides, enabling them to appreciate the nuances in choosing the most appropriate radionuclide for a given application. The comparison with other radioisotopes used in cancer therapy offers valuable context, showcasing the unique advantages that α-emitters bring to the table.
Mechanisms of action
Mechanisms of action delve into the radiobiology of α-particles and how they exert their effects on cancer cells.[14] This section discusses the high LET of α-particles, their interactions with biological tissues and the resulting deoxyribonucleic acid damage. The intricate processes by which α-particles cause irreparable harm to cancer cells, including double-strand breaks and complex lesions, are explored.[15] In addition, the immunomodulatory effects of α-therapy are considered, shedding light on the broader impact of this treatment approach on the immune system’s response to cancer.[16]
Preclinical studies
Preclinical studies are a critical bridge between laboratory research and clinical applications. This section delves into the use of animal models and in vitro experiments to assess the efficacy and safety of targeted α-therapy.[17] Readers will learn how researchers test the potential of α-emitters in various cancer models, gaining insights into the promising results and challenges encountered during this phase. The lessons from preclinical research are valuable in understanding the transition from laboratory success to clinical trials.
DISCUSSION
The preceding sections have taken us on a journey through the evolution and revolutionary potential of targeted α-therapy in the realm of clinical applications.[18] As we delve into the discussion, we will synthesise the key findings, assess the current state of α-therapy and contemplate its future in cancer treatment.
Efficacy and limitations in clinical trials
The clinical trials and applications of α-therapy have demonstrated both promise and challenges. While some studies have reported remarkable responses and disease control,[19-21] others have encountered hurdles such as limited patient populations,[22] variable treatment outcomes[23] and logistical complexities.[24,25] These trials underscore the importance of careful patient selection and the need for broader research to elucidate the factors influencing α-therapy efficacy.
Safety and toxicity management
The issue of safety and toxicity is a paramount concern in α-therapy. While the selective nature of α-particles minimises damage to healthy tissues, the potential for radiotoxicity in critical organs remains a challenge.[26] Ongoing efforts to develop radiopharmaceuticals that enhance safety profiles are promising, but rigorous patient monitoring and dosimetry calculations are vital components of successful α-therapy.[27]
Patient selection and imaging
Patient selection in α-therapy is evolving toward a personalised approach, capitalising on diagnostic imaging techniques for treatment planning. Advances in imaging technologies, such as positron emission tomography and single-photon emission computed tomography, allow for improved dosimetry calculations and precise targeting.[28-30] The integration of diagnostic imaging into treatment workflows represents a significant step in optimising α-therapy outcomes.
Future directions
The future of targeted α-therapy is brimming with possibilities. Combination therapies, where α-emitters are used in concert with other treatment modalities, have shown the potential to overcome resistance and improve overall therapeutic outcomes. Moreover, the exploration of α-therapy in non-oncological applications, such as immunomodulation and targeted radionuclide therapy for non-cancerous diseases, opens new horizons for this technology.
Regulatory and economic considerations
Regulatory approvals are pivotal for the widespread adoption of α-therapy. The role of agencies such as the Food and Drug Administration and international regulatory bodies cannot be underestimated, and the establishment of clear guidelines and standards is essential. In addition, economic factors, including the cost-effectiveness of α-therapy, access to treatment and insurance coverage, will influence its integration into mainstream oncology.
CONCLUSION
The clinical translation of targeted α-therapy is undoubtedly an evolution in the field of cancer treatment. While it may not yet constitute a full-blown revolution, the accumulated knowledge, ongoing research and growing body of evidence suggest that it has the potential to transform the landscape of oncology. However, for α-therapy to realise its full potential, multidisciplinary collaboration, further research, and diligent efforts to address safety and regulatory aspects are essential. The journey toward establishing α-therapy as a mainstream cancer treatment modality is a testament to the enduring human quest to conquer one of the most challenging diseases of our time.
Ethical approval
The Institutional Review Board approval is not required.
Declaration of patient consent
Patient’s consent is not required as patients’ identity is not disclosed or compromised.
Conflicts of interest
There are no conflicts of interest.
Use of artificial intelligence (AI)-assisted technology for manuscript preparation
The authors confirm that there was no use of artificial intelligence (AI)-assisted technology for assisting in the writing or editing of the manuscript and no images were manipulated using AI.
Financial support and sponsorship: Nil.
References
- Alpha Emitter Radium-223 and Survival in Metastatic Prostate Cancer. N Engl J Med. 2013;369:213-23.
- [CrossRef] [PubMed] [Google Scholar]
- Targeted α-particle Cancer Therapy. Annu Rev Med. 2007;58:221-34.
- [CrossRef] [PubMed] [Google Scholar]
- Radioimmunotherapy of Solid Tumors: The Promise of Targeted α-particle Radiation Therapy. J Nucl Med. 2005;46:199-204.
- [Google Scholar]
- Dose Escalation and Dosimetry of First-in-human Alpha Radioimmunotherapy with 212Pb-TCMC-Trastuzumab. J Nucl Med. 2014;55:1636-42.
- [CrossRef] [PubMed] [Google Scholar]
- 177Lu-labeled Prostate-Specific Membrane Antigen Targeting Peptide 6. Cancer Biother Radiopharm. 2018;33:13-7.
- [Google Scholar]
- Radium-223 in the Treatment of Osteoblastic Metastases: A Critical Clinical Review. Int J Radiat Oncol Biol Phys. 2015;91:898-906.
- [CrossRef] [PubMed] [Google Scholar]
- Radioimmunotherapy of Micrometastases in a Human Tumor Xenograft Model: Aspects of Re-treatment and the Treatment of Multiple Disease Sites. Radiat Res. 1997;148:S21-5.
- [CrossRef] [PubMed] [Google Scholar]
- A Review of Targeted Alpha-Particle Therapy in Oncology. Open Nucl Med J. 2019;11:23-33.
- [Google Scholar]
- Radioimmunotherapy of Breast Cancer Metastases with α-particle Emitter 225Ac: Comparing Efficacy with 213Bi and 90Y. Cancer Res. 2009;69:8941-8.
- [CrossRef] [PubMed] [Google Scholar]
- The Alpha-particle Immunotherapy: Victor or Vanquished. Cancer Biother Radiopharm. 2013;28:637-42.
- [Google Scholar]
- Effects of Resveratrol, Curcumin, Berberine and Other Nutraceuticals on Aging, Cancer Development, Cancer Stem Cells and microRNAs. Aging (Albany NY). 2017;9:1477-536.
- [CrossRef] [PubMed] [Google Scholar]
- Charged Particles in Radiation Oncology. Nat Rev Clin Oncol. 2010;7:37-43.
- [CrossRef] [PubMed] [Google Scholar]
- Nuclear Astrophysics Far from Stability: CEMP and r-Process Enhancement. Prog Part Nucl Phys. 2020;112:103770.
- [Google Scholar]
- Intraperitoneal alpha-particle radioimmunotherapy of ovarian cancer patients: Pharmacokinetics and dosimetry of (211)At-MX35 F(ab')2-a phase I study. J Nucl Med. 2009;50:1153-60.
- [CrossRef] [PubMed] [Google Scholar]
- Pharmacokinetics and Biodistribution of (86)Y-Trastuzumab for (90)Y Dosimetry in an Ovarian Carcinoma Model: Correlative MicroPET and MRI. J Nucl Med. 2003;44:1148-55.
- [Google Scholar]
- Intralesional Targeted Alpha-particle Radioimmunotherapy for Metastatic Melanoma. Cancer Biol Ther. 2005;4:1318-24.
- [CrossRef] [PubMed] [Google Scholar]
- Clinical Experience with Alpha-particle Emitting 211At: Treatment of Recurrent Brain Tumor Patients with 211At-labeled Chimeric Antitenascin Monoclonal Antibody 81C6. J Nucl Med. 2008;49:30-8.
- [CrossRef] [PubMed] [Google Scholar]
- Targeted Alpha-radionuclide Therapy of Functionally Critically Located Gliomas with 213Bi-DOTA-[Thi8,Met(O2)11]-Substance P: A Pilot Trial. Eur J Nucl Med Mol Imaging. 2010;37:1335-44.
- [CrossRef] [PubMed] [Google Scholar]
- Actinium-225 in Targeted Alpha-particle Therapeutic Applications. Curr Radiopharm. 2018;11:200-14.
- [Google Scholar]
- Cancer and radiation therapy: Current advances and future directions. Int J Med Sci. 2012;9:193-9.
- [CrossRef] [PubMed] [Google Scholar]
- Production of Ac-225 from Th-229 for Targeted Alpha-particle Cancer Therapy. Anal Chem. 2005;77:6288-91.
- [CrossRef] [PubMed] [Google Scholar]
- Radioimmunotherapy of Cancer with High Linear Energy Transfer (LET) Radiation Delivered by Radionuclides Emitting Alpha-particles or Auger Electrons. Adv Drug Deliv Rev. 2017;109:102-18.
- [CrossRef] [PubMed] [Google Scholar]
- Preclinical Evaluation of 203/212Pb-labeled Low-molecular-weight Compounds for Targeted Radiopharmaceutical Therapy of Prostate Cancer. J Nucl Med. 2014;55:1892-8.
- [Google Scholar]
- An Overview of Targeted Alpha-particle Therapies in Preclinical and Clinical Research. Int J Radiat Oncol Biol Phys. 2019;103:511-7.
- [Google Scholar]
- Current Clinical and Preclinical Alpha Particle Radiopharmaceuticals for Cancer Therapy. J Label Compd Radiopharm. 2021;64:700-22.
- [Google Scholar]
- MIRD Pamphlet No. 21: A Generalized Schema for Radiopharmaceutical Dosimetry-standardization of Nomenclature. J Nucl Med. 2009;50:477-84.
- [CrossRef] [PubMed] [Google Scholar]
- Mechanisms of Hematopoietic Effects of Ionizing Radiation Irradiation. Front Pharmacol. 2012;3:40.
- [Google Scholar]
- Intraperitoneal Alpha-particle Radioimmunotherapy of Ovarian Cancer Patients: Pharmacokinetics and Dosimetry of (211)At-MX35 F(ab')2-a Phase I Study. J Nucl Med. 2009;50:1153-60.
- [CrossRef] [PubMed] [Google Scholar]
- Bifunctional Chelates for Metal Nuclides. Q J Nucl Med Mol Imaging. 1994;38:159-66.
- [Google Scholar]